Understanding Friction in A-Level Maths Mechanics
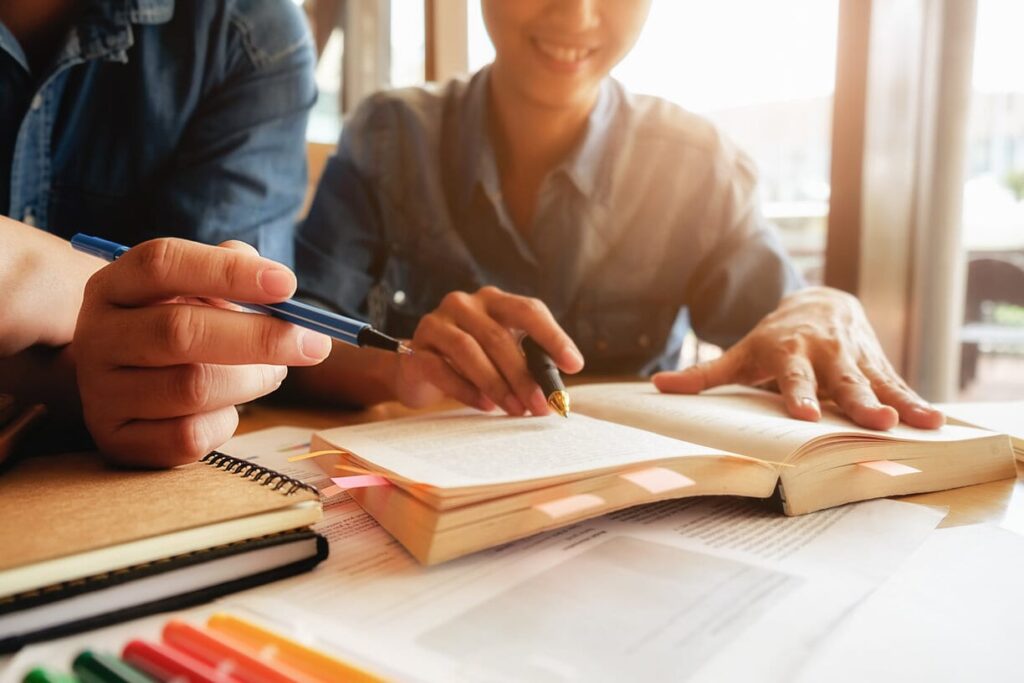
Mechanics – Introduction
Understanding friction in A-Level Maths Mechanics is a crucial topic that plays a significant role in various real-world applications. Friction is a force that opposes the motion of objects in contact with each other, and it is essential to comprehend its effects on different systems. By delving into the intricacies of friction, students can gain a deeper understanding of how forces interact in mechanical systems, which is fundamental in the field of engineering and physics.
Having a strong grasp of the concept of friction is paramount for A-Level Maths Mechanics students as it forms the basis for solving complex problems related to motion and forces. Without a thorough understanding of friction, students may struggle to analyse and predict the behaviour of objects in motion accurately. Moreover, mastering the concept of friction enables students to make informed decisions when designing structures or machinery, as frictional forces can significantly impact the performance and efficiency of these systems.
This blog post aims to provide readers with a comprehensive guide to understanding friction in A-Level Maths Mechanics. By breaking down the concept into manageable sections and offering practical examples, readers will be able to enhance their knowledge and skills in this area. With a clear explanation of the principles of friction and its applications, students can excel in their studies and approach challenging problems with confidence and proficiency.
What is Friction?
Friction is a fundamental concept in mechanics that refers to the force that opposes the relative motion or tendency of motion between two surfaces in contact. It plays a crucial role in various mechanical systems, as it affects the efficiency, stability, and control of objects in motion. Friction can be both beneficial and detrimental, depending on the context and application.
The occurrence of friction between two surfaces arises due to the microscopic irregularities present on their contact surfaces. When two objects come into contact, these irregularities interlock, creating resistance to motion. This interlocking mechanism is known as adhesion. Additionally, another type of friction called sliding friction occurs when the irregularities on the surfaces slide past each other, generating heat and reducing the motion between the objects.
Several factors influence the magnitude of friction between two surfaces. Firstly, the nature of the materials involved plays a significant role. Different materials have varying levels of roughness and adhesion, which directly impact the friction between them. For example, rough surfaces tend to have higher friction compared to smooth surfaces. Secondly, the normal force or the perpendicular force pressing the surfaces together affects friction. The greater the normal force, the stronger the frictional force. Thirdly, the type of motion, whether it is sliding, rolling, or fluid friction, also affects the magnitude of friction. Lastly, the presence of lubricants or contaminants between the surfaces can either increase or decrease friction, depending on their properties and the specific situation.
Types of Friction
Friction, a force that opposes motion, can be categorised into various types, including static friction, kinetic friction, and rolling friction. Static friction occurs when two surfaces are in contact but not moving relative to each other. It prevents objects from sliding or moving when a force is applied. On the other hand, kinetic friction arises when two surfaces are in motion relative to each other. It acts to slow down or stop the motion of objects. Lastly, rolling friction occurs when an object rolls over a surface, such as a ball rolling on the ground.
Each type of friction exhibits distinct characteristics and behaviours. Static friction is typically greater than kinetic friction, meaning it requires more force to overcome static friction and initiate motion. It depends on the nature of the surfaces in contact and can vary depending on factors like roughness and weight.
Kinetic friction, on the other hand, remains relatively constant once motion is initiated. It is generally lower than static friction and depends on the nature of the surfaces and the force pressing them together. Rolling friction is usually the smallest of the three types and occurs when an object rolls over a surface. It is influenced by factors like the shape of the object and the nature of the surface.
Real-life examples can help illustrate the application of these types of friction. Static friction can be observed when trying to push a heavy object, such as a car stuck in mud. The force required to overcome static friction is often greater than the force needed to keep the object in motion.
Kinetic friction can be experienced when sliding a book across a table. Initially, it requires more force to overcome static friction, but once the book is in motion, the force required to keep it moving is lower. Rolling friction is evident when a bicycle rolls on the road. The friction between the tires and the road surface allows the bike to move forward while also providing control and stability. Understanding these types of friction and their characteristics is crucial in various fields, including engineering, physics, and everyday life.
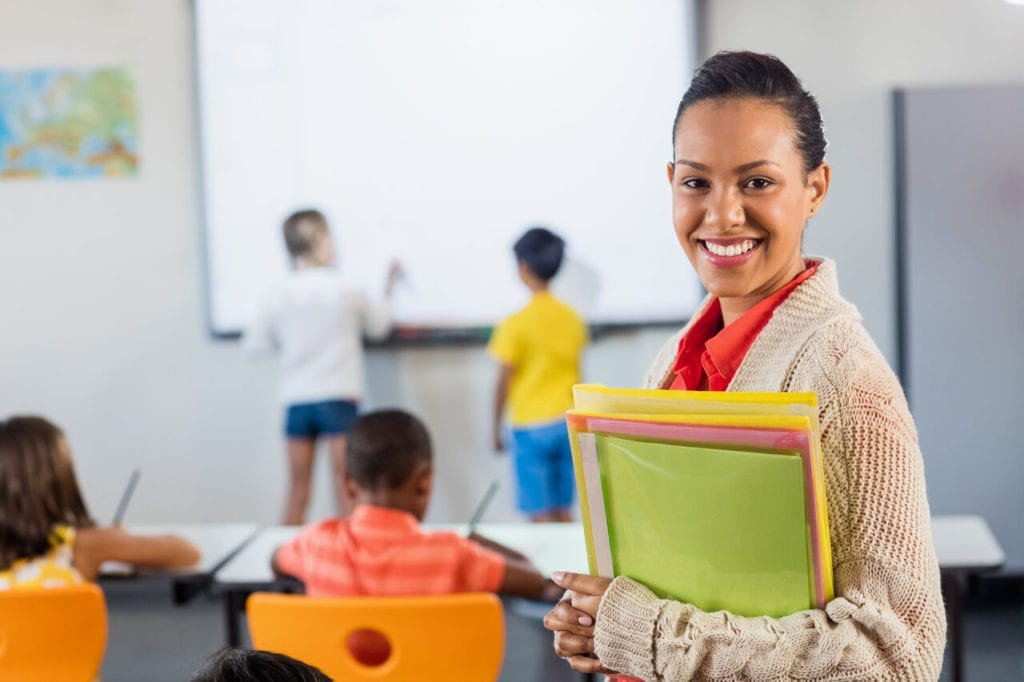
Laws of Friction
Newton’s laws of friction are fundamental principles that describe the behaviour of frictional forces between two surfaces in contact. The first law states that friction is directly proportional to the normal force acting on an object. This means that as the normal force increases, the frictional force also increases. The second law of friction states that the frictional force is independent of the contact area between the two surfaces.
This means that the amount of friction does not depend on how much surface area is in contact, but rather on the nature of the surfaces themselves. The third law of friction states that the direction of the frictional force is opposite to the direction of motion or impending motion of the object.
The relationship between frictional force, normal force, and coefficient of friction can be explained using the equation: F_friction = μ * F_normal. In this equation, F_friction represents the frictional force, F_normal represents the normal force, and μ represents the coefficient of friction. The coefficient of friction is a dimensionless quantity that represents the ratio of the frictional force to the normal force.
It is a property of the two surfaces in contact and can vary depending on factors such as surface roughness, material properties, and temperature. The normal force is the force exerted by a surface perpendicular to the contact surface, while the frictional force is the force that opposes the motion or impending motion of an object.
The mathematical formulas and equations related to friction include the equation for calculating the maximum static frictional force: F_friction(max) = μ_s * F_normal, where μ_s is the coefficient of static friction. Another important equation is the equation for calculating the kinetic frictional force: F_friction(kinetic) = μ_k * F_normal, where μ_k is the coefficient of kinetic friction.
These equations are essential for understanding and predicting the behaviour of frictional forces in various situations, such as sliding objects, rolling objects, and objects on inclined planes. By applying these formulas, engineers and scientists can design systems and structures that minimise frictional losses and optimise performance.
Coefficient of Friction
The coefficient of friction is a measure of the resistance to motion between two surfaces in contact. It quantifies the amount of frictional force that exists between the surfaces and is denoted by the symbol “μ”. The coefficient of friction is significant as it helps in understanding and predicting the behaviour of objects in contact. It plays a crucial role in various fields such as physics, engineering, and everyday life.
Calculating the coefficient of friction for different surfaces involves conducting experiments or using theoretical models. One common method is the inclined plane experiment, where a block is placed on an inclined plane and the angle at which it starts to slide is measured. By analysing the forces acting on the block, including the gravitational force and the normal force, the coefficient of friction can be determined using trigonometric equations.
Another method is the use of a friction tester, which applies a known force to a surface and measures the resulting frictional force. By dividing the measured frictional force by the applied force, the coefficient of friction can be calculated. Additionally, theoretical models based on the properties of the surfaces in contact can be used to estimate the coefficient of friction.
To reinforce understanding, let’s consider a few examples and practice problems. For instance, imagine a wooden block being pushed across a table. The coefficient of friction between the wood and the table determines how easy or difficult it is to move the block. If the coefficient of friction is high, it will require more force to move the block, while a low coefficient of friction will make it easier to slide the block.
In a practice problem, let’s say we have a 10 kg box placed on a horizontal surface. The box requires a force of 50 N to start moving. By dividing the force required to initiate motion (50 N) by the weight of the box (10 kg × 9.8 m/s²), we can calculate the coefficient of friction. In this case, the coefficient of friction would be 0.51.
By exploring various examples and solving practice problems, individuals can gain a better understanding of the coefficient of friction and its practical applications. This knowledge can be applied in fields such as designing machinery, analysing the performance of vehicles, and ensuring safety in everyday activities.
Applications of Friction in Mechanics
Friction plays a crucial role in various mechanical systems by providing resistance to motion between two surfaces in contact. In inclined planes, friction helps to prevent objects from sliding down the slope uncontrollably. Without friction, objects would slide down the incline at a constant speed, making it difficult to control their movement. By increasing the friction between the object and the surface of the incline, the speed at which the object descends can be controlled, allowing for a more predictable and manageable motion.
In pulley systems, friction between the rope and the pulley wheels helps to transfer force from one end of the system to the other. The friction between the rope and the pulley wheels allows for the tension in the rope to be maintained, ensuring that the system operates smoothly and efficiently. Without friction, the rope would slip on the pulley wheels, causing the system to fail to transfer force effectively. Therefore, friction is essential in ensuring the proper functioning of pulley systems.
Friction also plays a significant role in circular motion by providing the centripetal force necessary to keep an object moving in a circular path. When an object moves in a circle, the friction between the object and the surface it is moving on provides the centripetal force required to keep the object in its curved path. Without friction, the object would continue in a straight line, unable to change its direction and maintain circular motion. Therefore, friction is essential in enabling objects to move in circular paths and maintain equilibrium in their motion.
Frictional Forces and Motion
Frictional forces play a crucial role in influencing the motion of objects. When two surfaces come into contact, friction occurs due to the interlocking of microscopic irregularities. This force opposes the relative motion between the surfaces and can have significant effects on an object’s acceleration, velocity, and displacement.
Friction affects acceleration by acting in the opposite direction to the applied force. When an object is pushed or pulled, the frictional force acts in the opposite direction, reducing the net force and thus affecting the object’s acceleration. For example, when a car accelerates, the friction between the tires and the road surface opposes the forward motion, making it harder for the car to accelerate.
Friction also impacts velocity by reducing it over time. As an object moves, the frictional force acts in the opposite direction to its motion, gradually slowing it down. This is evident when a ball rolls on a rough surface. Initially, the ball’s velocity decreases due to the frictional force until it eventually comes to a stop.
In terms of displacement, friction can limit the distance an object can travel. For instance, if a book is pushed along a table, the friction between the book and the table surface opposes its motion, resulting in a shorter displacement compared to a scenario with minimal friction.
To further illustrate the impact of friction on motion, let’s consider a few examples. Imagine a person sliding a heavy box across a rough floor. The frictional force between the box and the floor opposes the person’s applied force, making it more difficult to move the box. This demonstrates how friction affects acceleration by reducing the rate at which the box accelerates.
Another example is a car driving on a wet road. The water on the road surface increases the friction between the tires and the road, resulting in a decrease in the car’s velocity. This decrease in velocity is due to the increased frictional force acting against the car’s forward motion.
Now, let’s visualise these concepts with diagrams. Consider a block resting on an inclined plane. When a force is applied to the block, the frictional force acts in the opposite direction, opposing the motion. A diagram can show the force vectors, with the applied force pointing downwards and the frictional force pointing upwards. This visual representation helps to understand how friction affects the motion of the block.
Overcoming Friction
Strategies to overcome friction in mechanical systems involve various approaches that aim to minimise the resistance between moving parts. One effective strategy is to use lubrication, which involves the application of a lubricant between the surfaces in contact. Lubricants can be in the form of oils, greases, or solid films, and they work by creating a thin layer that separates the surfaces, reducing direct contact and friction. This not only helps in reducing wear and tear but also enhances the overall efficiency and performance of the mechanical system.
Lubrication plays a crucial role in reducing friction in mechanical systems. By providing a lubricant between the moving parts, it forms a protective layer that prevents direct metal-to-metal contact. This layer acts as a barrier, reducing the frictional forces and allowing the parts to move smoothly. Lubrication also helps in dissipating heat generated due to friction, preventing overheating and potential damage to the system. Additionally, lubricants can also act as a sealant, preventing the entry of contaminants and moisture that could further increase friction and cause corrosion.
While reducing friction offers several advantages, it is important to consider the specific scenarios in which it is applied. In some cases, such as in high-precision machinery or delicate instruments, minimising friction is crucial to ensure accurate and precise movements. Reduced friction also leads to less energy loss, making mechanical systems more efficient and cost-effective. However, there are also scenarios where friction is intentionally increased, such as in braking systems, where the friction between brake pads and rotors is necessary to slow down or stop a vehicle.
It is essential to strike a balance between reducing friction for improved performance and maintaining sufficient friction for safety and control. Additionally, excessive reduction of friction can lead to issues like slippage or loss of traction, which can be detrimental in certain applications like vehicle tires or industrial machinery. Therefore, careful consideration of the advantages and disadvantages of reducing friction is necessary to optimise the performance and safety of mechanical systems in different scenarios.
Practical Applications of Understanding Friction
Understanding friction is crucial in a wide range of real-world applications across various fields. Friction plays a significant role in industries such as automotive, aerospace, and manufacturing, where engineers and designers rely on this force to enhance efficiency and safety in their designs. By comprehending how friction works and how it can be manipulated, professionals in these industries can develop innovative solutions that improve the performance and reliability of their products.
Engineers and designers leverage friction in numerous ways to optimise the functionality of their creations. For instance, in the automotive industry, tire manufacturers engineer treads with specific patterns to maximise friction between the tire and the road surface, thereby improving traction and overall vehicle control.
In the field of biomechanics, prosthetic limb designers utilise friction to ensure a secure and stable connection between the artificial limb and the user’s body, enhancing mobility and comfort. By strategically incorporating friction into their designs, these professionals can achieve higher levels of efficiency and safety in their products.
There are several examples of friction-related innovations and technologies that have revolutionised various industries. One notable example is the development of anti-lock braking systems (ABS) in vehicles, which utilise controlled friction to prevent skidding and improve braking performance on slippery surfaces.
Another example is the use of friction stir welding in aerospace manufacturing, a technique that creates strong and durable bonds between metal components without the need for traditional welding methods. These innovations highlight the importance of understanding and harnessing friction to drive advancements in technology and improve safety standards across different sectors.
Conclusion
In the blog post, several key points were discussed regarding the topic of friction in A-Level Maths Mechanics. The post highlighted the significance of understanding friction and its role in various mechanical systems. It explained how friction affects the motion of objects and the importance of considering it in mathematical calculations.
Understanding friction in A-Level Maths Mechanics is of utmost importance. Friction is a force that opposes the motion of objects and plays a crucial role in determining the behaviour of mechanical systems. By comprehending the concept of friction, students can accurately analyse and predict the motion of objects in real-world scenarios. It allows them to solve complex problems involving forces, motion, and equilibrium, providing a solid foundation for further studies in physics and engineering.
To excel in A-Level Maths Mechanics, it is essential for readers to continue exploring and practising the concepts covered in the guide. By delving deeper into the topic of friction, students can gain a more comprehensive understanding of its applications and implications. They can further enhance their problem-solving skills by attempting various exercises and problems related to friction.
Continuous practice and exploration will not only strengthen their knowledge but also enable them to tackle more advanced topics in mechanics with confidence. So, keep exploring, practising, and embracing the importance of understanding friction in A-Level Maths Mechanics.