A Level Maths Mechanics: 10 Tricks You Need to Know
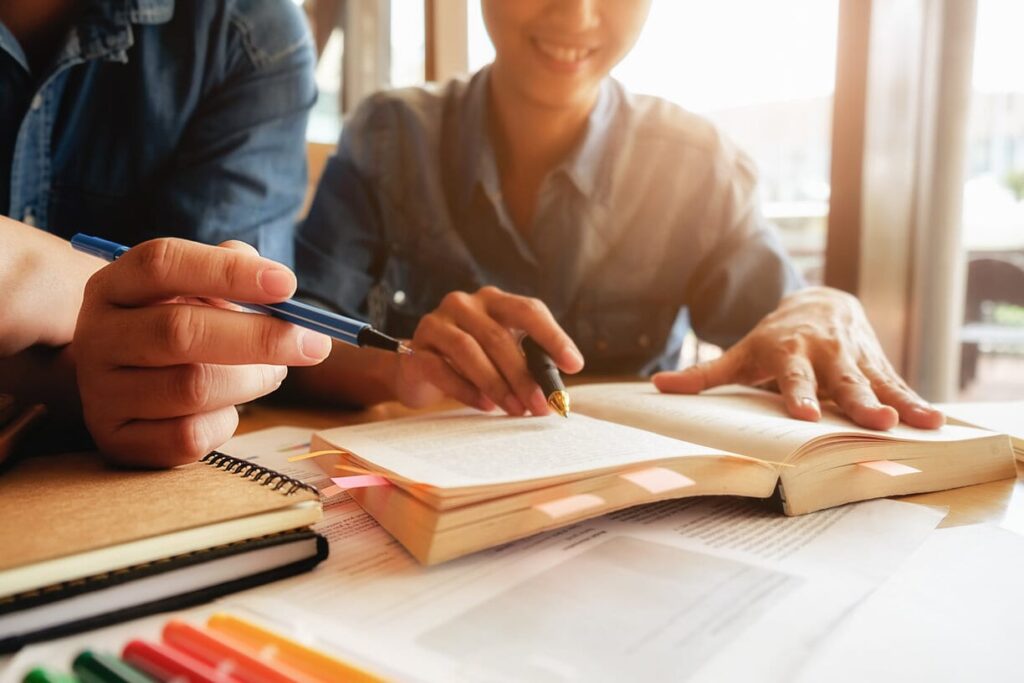
A Level Maths Mechanics – Introduction
A Level Maths Mechanics is a crucial component of the curriculum that focuses on the study of motion, forces, and energy. It delves into the principles and laws that govern the physical world, providing students with a deeper understanding of how objects move and interact. This subject plays a vital role in various fields such as engineering, physics, and even everyday life, as it equips students with problem-solving skills and analytical thinking.
Despite its significance, many students encounter challenges when studying A Level Maths Mechanics. One common difficulty is grasping the abstract concepts and mathematical formulas involved.
Understanding the principles of motion and forces can be complex, requiring students to visualise and apply mathematical equations to real-world scenarios. Additionally, the subject often demands a strong foundation in algebra and calculus, which can pose difficulties for those who struggle with these mathematical concepts.
The purpose of this blog post is to provide students with 10 essential tricks to excel in A Level Maths Mechanics. By offering practical tips and strategies, the aim is to help students overcome the challenges they may face and enhance their understanding of the subject.
These tricks may include breaking down complex problems into smaller, manageable steps, practising with a variety of examples, and seeking additional resources such as textbooks or online tutorials. Ultimately, the blog post aims to empower students with the tools and techniques necessary to succeed in A Level Maths Mechanics and achieve their academic goals.
Trick 1: Understanding Vectors
Vectors play a crucial role in mechanics as they are used to represent physical quantities such as displacement, velocity, and force. In mechanics, vectors are essential for describing the direction and magnitude of these quantities, which are necessary for solving problems related to motion and forces.
Understanding vectors is important because they allow us to analyse and predict the behaviour of objects in motion. By using vectors, we can break down complex physical phenomena into simpler components, making problem-solving more manageable and efficient.
To effectively understand and manipulate vectors, it is important to grasp the concept of vector addition and subtraction. Vectors can be added or subtracted by breaking them down into their components along the x and y axes.
It is also crucial to understand vector multiplication, including scalar multiplication and the dot product. Practice drawing vectors on a coordinate system and visualising their direction and magnitude. Additionally, familiarise yourself with vector notation and terminology to communicate vector quantities accurately.
Common mistakes students make when dealing with vectors in mechanics include confusing vector quantities with scalar quantities, neglecting the direction of vectors, and incorrectly applying vector operations. To avoid these mistakes, always pay attention to the direction of vectors and ensure that they are added or subtracted correctly.
Practice solving a variety of vector problems to build proficiency and confidence in manipulating vectors. Seek help from teachers, tutors, or online resources if you encounter difficulties in understanding vector concepts. By practising regularly and seeking clarification when needed, you can improve your skills in working with vectors effectively in mechanics.
Trick 2: Resolving Forces
Resolving forces is a fundamental concept in mechanics that involves breaking down a force into its individual components in order to analyse its effects on an object. This concept is based on the understanding that a force can act in different directions and can be decomposed into two or more forces acting along different axes. By resolving forces, we can determine the magnitude and direction of each component force, which allows us to accurately calculate the resulting effects on an object.
To resolve forces accurately, follow these step-by-step instructions. Firstly, identify the force that needs to be resolved and determine the axes along which it will be resolved. Next, draw a clear and accurate diagram representing the force and its direction. Then, choose a suitable coordinate system and assign positive and negative directions to each axis.
Now, using trigonometric principles, break down the force into its components along each axis. This can be done by using the sine and cosine functions to calculate the magnitudes of the components. Finally, calculate the resultant forces along each axis by summing up the individual components. This will provide you with the accurate resolved forces.
Common misconceptions related to resolving forces can arise from a lack of understanding or confusion about the concept. One common misconception is that resolving forces changes the magnitude of the force. However, it is important to note that resolving forces only breaks down a force into its components and does not alter its magnitude. Another misconception is that resolving forces is only applicable to forces acting at an angle. In reality, resolving forces can be applied to any force, regardless of its direction.
To overcome these misconceptions, it is crucial to emphasise the fundamental principles of resolving forces. Providing clear explanations and examples can help students grasp the concept more effectively. Additionally, practising various scenarios and exercises that involve resolving forces can enhance understanding and accuracy.
Encouraging critical thinking and problem-solving skills can also help students overcome misconceptions by allowing them to analyse and apply the concept in different contexts. By addressing these common misconceptions and providing proper guidance, students can develop a solid understanding of resolving forces and its application in mechanics.
Trick 3: Free Body Diagrams
Free body diagrams play a crucial role in mechanics problem-solving as they provide a visual representation of the forces acting on an object. By breaking down a complex system into individual forces, free body diagrams allow us to analyse and understand the forces at play in a given situation. This understanding is essential for solving mechanics problems accurately and efficiently.
To construct accurate and clear free body diagrams, there are several guidelines that can be followed. Firstly, it is important to identify all the forces acting on the object and represent them as vectors. These forces can include gravitational forces, normal forces, frictional forces, and applied forces. Each force should be labelled with its magnitude and direction.
Additionally, it is crucial to choose a suitable coordinate system and clearly indicate it on the diagram. This helps in accurately representing the direction of forces and simplifies the calculations involved. The diagram should also include the object of interest, clearly indicating its shape and dimensions.
Furthermore, it is important to ensure that the free body diagram is neat and organised. The forces should be drawn as arrows, with the length of the arrow representing the magnitude of the force. The arrows should be labelled with the corresponding force and its value. By following these guidelines, accurate and clear free body diagrams can be constructed.
Common errors that students make when constructing free body diagrams include neglecting certain forces, incorrectly representing the direction of forces, and failing to label the forces accurately. To improve their diagramming skills, students should pay attention to the following aspects.
Firstly, it is important to consider all the forces acting on the object, including those that may be easily overlooked, such as frictional forces or tension forces. By carefully analysing the situation, students can ensure that all relevant forces are included in the free body diagram.
Secondly, students should be cautious when representing the direction of forces. It is common for students to confuse the direction of forces, especially when dealing with inclined planes or complex systems. Double-checking the direction of forces and referring to the problem statement can help avoid this error.
Lastly, accurate labelling of forces is crucial. Students should clearly indicate the magnitude and direction of each force, ensuring that the labels are legible and placed appropriately on the diagram. This helps in avoiding confusion and ensures that the diagram accurately represents the forces at play.
By being mindful of these common errors and following the guidelines mentioned earlier, students can improve their diagramming skills and construct accurate and clear free body diagrams, ultimately enhancing their problem-solving abilities in mechanics.
Trick 4 – Newton’s Laws
Newton’s laws of motion are a set of fundamental principles that describe the behaviour of objects in motion. The first law, also known as the law of inertia, states that an object at rest will remain at rest, and an object in motion will continue moving at a constant velocity unless acted upon by an external force. This law emphasises the concept of inertia, which is the tendency of an object to resist changes in its motion.
The second law of motion states that the acceleration of an object is directly proportional to the net force applied to it and inversely proportional to its mass. This law can be mathematically expressed as F = ma, where F represents the net force, m represents the mass of the object, and a represents the acceleration. By understanding this law, one can effectively calculate the force required to accelerate or decelerate an object.
The third law of motion states that for every action, there is an equal and opposite reaction. This means that whenever an object exerts a force on another object, the second object exerts an equal and opposite force on the first object. This law is particularly important in understanding the concept of forces in pairs, where two objects interact with each other by exerting equal and opposite forces.
When applying Newton’s laws of motion in mechanics problems, it is crucial to identify the forces acting on the object and determine their magnitudes and directions. It is also important to consider the mass of the object and the resulting acceleration. By carefully analysing the problem and applying the appropriate laws, one can effectively solve mechanics problems.
However, there are common pitfalls that one should be aware of when applying Newton’s laws of motion. One common mistake is neglecting certain forces or incorrectly identifying the forces acting on the object. It is important to consider all the forces, including friction, tension, and gravitational forces, to accurately analyse the problem.
Another pitfall is failing to consider the mass of the object and its impact on the acceleration. Neglecting the mass or using incorrect values can lead to inaccurate results. To avoid these pitfalls, it is essential to carefully read and understand the problem, identify all the forces involved, and apply the appropriate laws of motion accurately.
Trick 5: Conservation of Energy
The concept of conservation of energy is a fundamental principle in mechanics that states that energy cannot be created or destroyed, but only transferred or transformed from one form to another. This principle is based on the law of conservation of energy, which is a fundamental law of physics. In simple terms, it means that the total amount of energy in a closed system remains constant over time.
Energy conservation is highly relevant in mechanics as it allows us to analyse and understand the behaviour of objects and systems. By applying the principle of conservation of energy, we can determine the initial and final states of a system, calculate the work done, and predict the motion of objects. It provides a powerful tool for solving problems related to mechanical systems, such as collisions, pendulums, and springs.
To solve energy conservation problems, there are several techniques and examples that can be employed. One common technique is to identify the different forms of energy involved in the system and track their transformations. For example, in a simple pendulum, the energy can be divided into potential energy (due to the height of the pendulum bob) and kinetic energy (due to the motion of the bob). As the pendulum swings, the energy is continuously transferred between these two forms.
Another technique is to apply the principle of conservation of mechanical energy, which states that the total mechanical energy (sum of kinetic and potential energy) remains constant in the absence of external forces like friction. This principle can be used to solve problems involving objects moving in conservative force fields, such as gravitational or spring forces.
When it comes to common mistakes made by students in understanding energy conservation, one of the most common errors is neglecting certain forms of energy or failing to account for all the energy transformations in a system. Students may overlook factors such as friction, air resistance, or non-conservative forces, which can lead to inaccurate calculations or predictions.
To enhance students’ understanding of energy conservation, it is important to emphasise the importance of considering all forms of energy and their transformations in a system. Providing real-life examples and practical applications can also help students grasp the concept better. Engaging students in hands-on activities, such as experiments or simulations, can further enhance their understanding by allowing them to observe and analyse energy transformations firsthand. Additionally, encouraging critical thinking and problem-solving skills can help students identify and rectify their mistakes, leading to a deeper understanding of the concept of conservation of energy in mechanics.
Trick 6: Projectile Motion
Projectile motion is a fundamental concept in physics that describes the motion of an object thrown into the air and influenced only by gravity. When an object is launched into the air at an angle, it follows a curved path known as a projectile trajectory.
The motion can be broken down into horizontal and vertical components, with the horizontal component remaining constant and the vertical component being affected by gravity. Understanding projectile motion is crucial in mechanics as it helps in predicting the trajectory of objects such as projectiles, rockets, and even sports balls.
When analysing and solving projectile motion problems, it is important to first identify the initial velocity, launch angle, and height of the object. By breaking down the motion into horizontal and vertical components, one can use equations of motion to calculate the time of flight, maximum height, range, and velocity at any given point. It is also helpful to draw a diagram illustrating the motion of the object and label the relevant variables. Additionally, utilising trigonometric functions such as sine and cosine can help in determining the components of velocity and acceleration in different directions.
Common misconceptions in projectile motion problems include neglecting air resistance, assuming the horizontal and vertical motions are independent, and overlooking the effects of initial velocity and launch angle. To overcome these misconceptions, it is important to consider all factors that may affect the motion of the object, including air resistance and external forces.
It is also crucial to remember that the horizontal and vertical motions are interconnected, and changes in one component will affect the other. By practising various problems and understanding the underlying principles of projectile motion, one can overcome these misconceptions and develop a deeper understanding of the concept.
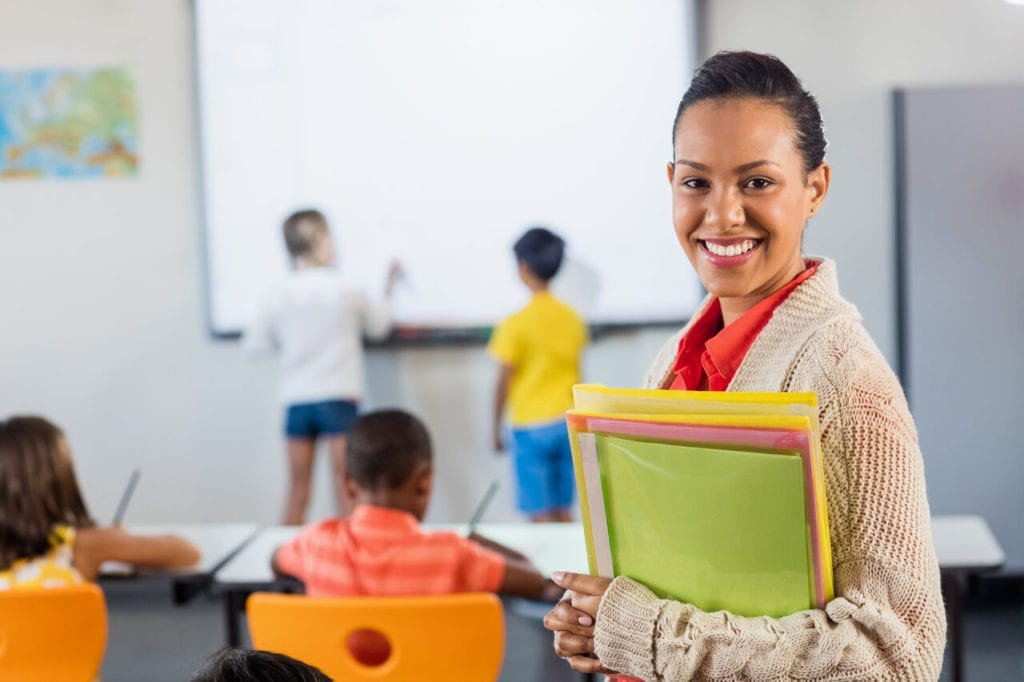
Trick 7: Moments and Torque
Moments and torque are fundamental concepts in mechanics that help us understand the rotational motion of objects. A moment is a measure of the turning effect of a force around a point or axis. It is calculated by multiplying the magnitude of the force by the perpendicular distance from the point or axis of rotation. Torque, on the other hand, is the rotational equivalent of force and is calculated by multiplying the force applied to an object by the perpendicular distance from the axis of rotation.
To calculate and manipulate moments effectively, it is important to consider the following tips. Firstly, choose a suitable point or axis of rotation to simplify the calculations. This point should be strategically selected to minimise the number of forces acting on the object. Secondly, accurately measure the perpendicular distance between the point of rotation and the line of action of the force.
This distance is crucial in determining the moment. Thirdly, ensure that the forces and distances are in the correct units, such as Newtons for force and metres for distance, to obtain accurate results. Lastly, when manipulating moments, remember that the sum of the clockwise moments is equal to the sum of the anticlockwise moments, as long as the object is in equilibrium.
Students often make common errors when dealing with moments and torque. One common mistake is miscalculating the perpendicular distance between the point of rotation and the line of action of the force. This can lead to incorrect moment calculations and inaccurate results. To improve understanding, students should practise accurately measuring distances and double-checking their calculations.
Another error is neglecting the direction of the moment. Moments can be either clockwise or anticlockwise, and it is important to consider their direction when calculating and manipulating them. Students should pay attention to the signs of the moments and ensure they are consistent throughout their calculations. Additionally, students may struggle with visualising the concept of moments and torque. Engaging in hands-on activities, such as using a lever or a see-saw, can help students develop a better understanding of how forces and distances affect rotational motion.
Trick 8: Circular Motion
Circular motion is a fundamental concept in mechanics that involves an object moving along a circular path at a constant speed. The principles of circular motion revolve around the idea that there is a centripetal force acting towards the centre of the circle, which keeps the object moving in a curved trajectory.
This force is necessary to counteract the natural tendency of an object to move in a straight line. Understanding the principles of circular motion is crucial in mechanics as it helps in analysing the motion of objects in various scenarios, such as the motion of planets around the sun or the movement of a car around a curve.
When it comes to analysing and solving circular motion problems, there are several techniques that can be employed. One common approach is to use Newton’s laws of motion to determine the net force acting on the object in circular motion. By setting up equations that account for the centripetal force and any other forces present, one can solve for the unknown variables such as the speed of the object or the radius of the circular path.
Another technique involves using the concept of angular velocity and acceleration to describe the rotational motion of the object in circular motion. By understanding these techniques, one can effectively analyse and solve complex circular motion problems.
Despite the straightforward nature of circular motion, there are common pitfalls that students may encounter when dealing with such problems. One common mistake is forgetting to consider all the forces acting on the object, leading to incorrect solutions. Another pitfall is misunderstanding the relationship between speed, radius, and centripetal force, which can result in errors in calculations.
To approach these pitfalls, it is important to carefully identify all the forces at play, draw clear diagrams to visualise the problem, and double-check calculations to ensure accuracy. By being aware of these common pitfalls and adopting a systematic approach to problem-solving, one can effectively tackle circular motion problems in mechanics.
Trick 9: Impulse and Momentum
Impulse and momentum are fundamental concepts in mechanics that help us understand the motion of objects. Impulse refers to the change in momentum of an object, and it is defined as the product of the force applied to an object and the time interval over which the force acts.
In simpler terms, impulse is the force applied to an object for a certain amount of time, resulting in a change in its momentum. On the other hand, momentum is the product of an object’s mass and its velocity. It is a vector quantity, meaning it has both magnitude and direction.
To solve impulse and momentum problems accurately, it is important to follow a systematic approach. Firstly, identify the given information such as the mass of the object, its initial velocity, and the force applied. Next, determine the time interval over which the force acts.
Once you have all the necessary information, you can calculate the impulse by multiplying the force and the time interval. To find the final velocity of the object, use the equation that relates impulse, mass, initial velocity, and final velocity. It is crucial to pay attention to the signs of the values, as they indicate the direction of the momentum.
Students often make common mistakes when solving impulse and momentum problems. One common mistake is neglecting the direction of the momentum. Momentum is a vector quantity, so it is important to consider both magnitude and direction. Another mistake is not properly identifying the given information and using the correct equations.
It is essential to carefully read the problem and identify the relevant variables. To enhance problem-solving skills, students can practise solving a variety of impulse and momentum problems. They can also seek clarification from their teachers or classmates if they are unsure about any concepts or steps. Additionally, visualising the problem and drawing diagrams can help in understanding and solving the problems accurately.
Trick 10: Systematic Approach to Problem-Solving
A systematic approach is crucial when it comes to solving mechanics problems. By following a structured methodical process, engineers and technicians can effectively analyse and address complex issues related to machinery, equipment, and systems. This approach involves breaking down the problem into smaller, more manageable parts, identifying the root cause of the issue, and systematically testing potential solutions to determine the most effective course of action.
Without a systematic approach, mechanics problem-solving can become chaotic and inefficient. By taking a haphazard or random approach to troubleshooting, individuals may overlook key details or make incorrect assumptions that can lead to further complications. In contrast, a systematic approach ensures that all relevant factors are considered, all possible solutions are explored, and the most appropriate solution is implemented in a logical and organised manner.
In conclusion, the importance of a systematic approach in mechanics problem-solving cannot be overstated. By following a structured and methodical process, individuals can effectively diagnose and resolve issues in a timely and efficient manner. This approach not only helps to ensure that problems are addressed accurately and comprehensively but also promotes a deeper understanding of the underlying principles and mechanisms at play in the field of mechanics.